Introduction to Quantum Computing and Cybersecurity
Quantum computing represents a significant leap in computational power, leveraging the principles of quantum mechanics to perform complex calculations at unprecedented speeds. In the realm of cybersecurity, this advancement poses both opportunities and threats. Traditional cybersecurity measures rely heavily on encryption algorithms that are secure against classical computers. However, the advent of quantum computing threatens to undermine these systems. For instance, Google’s quantum computer, Sycamore, achieved quantum supremacy by solving a problem in 200 seconds that would take the world’s fastest supercomputer 10,000 years. This section will provide an overview of quantum computing, its basic principles, and the current state of cybersecurity, setting the stage for understanding how quantum computing can both challenge and enhance cybersecurity measures.
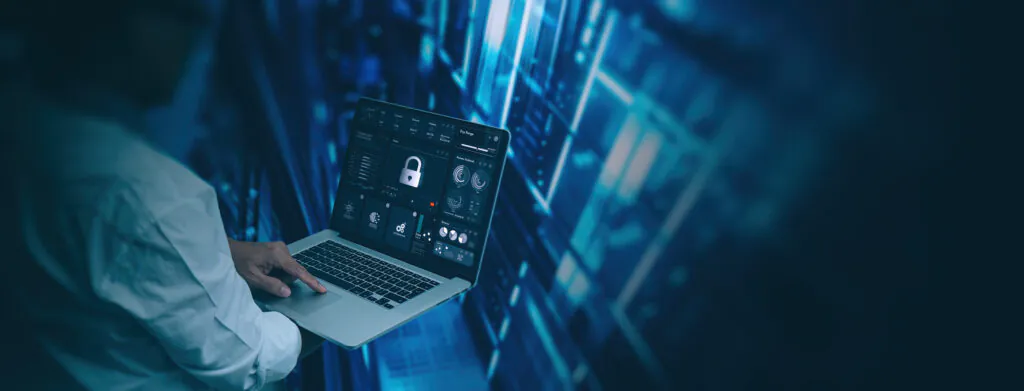
Quantum Computing: A New Paradigm
Quantum computing operates on the principles of superposition and entanglement, allowing quantum bits (qubits) to exist in multiple states simultaneously. This capability enables quantum computers to solve problems that are currently intractable for classical computers. For example, IBM’s quantum computer, IBM Q, is being used to explore new materials and drugs by simulating molecular structures that are too complex for classical computers. This section will delve into the fundamental differences between classical and quantum computing, explaining concepts such as qubits, quantum gates, and quantum algorithms. By understanding these differences, readers will appreciate the transformative potential of quantum computing and its implications for various fields, including cybersecurity.
Quantum Threats to Current Cryptographic Systems
One of the most significant threats posed by quantum computing is its ability to break traditional cryptographic systems. Algorithms such as RSA and ECC, which underpin much of today’s secure communication, rely on the difficulty of factoring large numbers or solving discrete logarithms—tasks that quantum computers can perform efficiently using Shor’s algorithm. For instance, a sufficiently powerful quantum computer could decrypt secure communications, exposing sensitive information. This section will explore how quantum computing can compromise these cryptographic systems, providing examples of vulnerable algorithms and discussing the potential consequences for data security and privacy.
Quantum-Resistant Cryptography
In response to the quantum threat, researchers are developing quantum-resistant cryptographic algorithms designed to withstand attacks from quantum computers. These algorithms, often based on lattice-based, hash-based, or code-based cryptography, aim to provide security even in a post-quantum world. For example, the National Institute of Standards and Technology (NIST) is leading an initiative to standardize quantum-resistant cryptographic algorithms. This section will discuss the development of these quantum-resistant algorithms, highlighting current research and advancements. It will also address the challenges of transitioning to these new cryptographic standards and the importance of proactive measures in safeguarding digital information.
Quantum Key Distribution (QKD)
Quantum Key Distribution (QKD) is a revolutionary method for secure communication that leverages the principles of quantum mechanics. Unlike classical key distribution methods, QKD ensures that any attempt to eavesdrop on the key exchange process will be detected, providing an unprecedented level of security. For instance, China’s Micius satellite has successfully demonstrated QKD over a distance of 1,200 kilometers, showcasing the potential for secure global communication. This section will explain how QKD works, including protocols like BB84 and E91, and discuss the benefits and challenges of implementing QKD in real-world scenarios. It will also explore the current state of QKD technology and its potential to enhance cybersecurity.
CASE STUDY
Case Study: Quantum Computing Enhancing Cybersecurity
Background
Quantum computing, leveraging the principles of quantum mechanics, offers unprecedented computational power. This capability is particularly transformative for cybersecurity, where traditional encryption methods face significant threats from quantum algorithms capable of breaking them.
The Challenge
Traditional cryptographic methods, such as RSA and ECC (Elliptic Curve Cryptography), rely on the difficulty of factoring large numbers or solving discrete logarithms. Quantum computers, with their ability to perform complex calculations exponentially faster than classical computers, pose a threat to these encryption techniques. This potential vulnerability necessitates the development of quantum-resistant cryptographic methods to secure sensitive data.
Implementation
One notable implementation of quantum computing in cybersecurity is Quantum Key Distribution (QKD). QKD uses the principles of quantum mechanics to securely exchange encryption keys between parties. Unlike classical key distribution methods, QKD can detect any eavesdropping attempts, ensuring the integrity and confidentiality of the keys.
For instance, the Chinese Micius satellite has successfully demonstrated QKD over long distances, enabling secure communication between ground stations thousands of kilometers apart. This implementation showcases the potential of quantum technologies to enhance secure communications on a global scale.
Benefits
Enhanced Security: Quantum computing enables the generation of truly random numbers, which are fundamental for secure cryptographic keys. This randomness is far superior to pseudo-random number generators used in classical computing, reducing the risk of key compromise.
Tamper Detection: QKD provides inherent tamper detection capabilities. Any attempt to intercept or measure the quantum keys alters their state, alerting the communicating parties to the presence of an eavesdropper.
Future-Proofing: By adopting quantum-resistant cryptographic methods, organizations can safeguard their data against future quantum threats. This proactive approach ensures long-term data security as quantum computing technology continues to evolve.
Case Study Example
The financial sector, with its high demand for secure transactions, has been an early adopter of quantum-enhanced cybersecurity measures. For example, JPMorgan Chase has partnered with IBM to explore quantum computing applications in cybersecurity. They are investigating how quantum algorithms can improve encryption methods and secure sensitive financial data against potential quantum attacks.
Conclusion
Quantum computing holds the promise of revolutionizing cybersecurity by providing robust, future-proof solutions to protect sensitive information. Through implementations like QKD and partnerships between tech companies and financial institutions, the cybersecurity landscape is being reshaped to address the challenges posed by quantum advancements.
Future Prospects and Challenges
The future of quantum computing in cybersecurity is both promising and uncertain. While quantum computing has the potential to revolutionize cybersecurity, significant challenges remain in terms of scalability, error correction, and integration with existing systems. For instance, Google’s quantum computer, Sycamore, still faces challenges in maintaining qubit coherence and error rates
-
Threat to Traditional Cryptography
Quantum computers have the potential to break widely used cryptographic algorithms such as RSA and ECC, which rely on the difficulty of factoring large numbers or solving discrete logarithms. Quantum algorithms like Shor’s algorithm can solve these problems exponentially faster than classical computers, posing a significant threat to current encryption methods.
-
Development of Quantum-Resistant Algorithms
To counter the threat posed by quantum computing, there is a need to develop and implement quantum-resistant algorithms. These algorithms are designed to be secure against quantum attacks, but their development and standardization are still in progress. Ensuring these new algorithms are robust and widely adopted is a significant challenge.
-
High Computational Requirements
Quantum computing requires substantial computational resources and sophisticated infrastructure. The development and maintenance of quantum computers are expensive and complex, which can limit their accessibility and widespread use. This high barrier to entry can slow down the progress in developing quantum-resistant cybersecurity measures.
-
Integration with Existing Systems
Integrating quantum-resistant algorithms and technologies into existing cybersecurity frameworks is a complex task. It requires significant changes to current systems and protocols, which can be time-consuming and costly. Ensuring compatibility and seamless integration without disrupting existing operations is a major challenge.
-
Data Security During Transition
As organizations transition to quantum-resistant cryptographic methods, there is a risk of data breaches and vulnerabilities. Ensuring data security during this transition period is crucial, as any weaknesses could be exploited by attackers using quantum computing capabilities.
-
Regulatory and Standardization Issues
The regulatory landscape for quantum computing and quantum-resistant cryptography is still evolving. Establishing global standards and regulations to ensure the secure implementation of quantum technologies is essential but challenging. Different countries may have varying approaches, complicating the development of a unified framework.
-
Limited Expertise
There is a shortage of experts in quantum computing and quantum-resistant cryptography. Developing and implementing secure quantum technologies requires specialized knowledge and skills, which are currently in limited supply. This expertise gap can hinder the progress in enhancing cybersecurity with quantum computing.
Ethical and Regulatory Considerations
The integration of quantum computing into cybersecurity raises important ethical and regulatory questions. Issues such as data privacy, surveillance, and the potential misuse of quantum technologies must be carefully considered. For example, the European Union’s General Data Protection Regulation (GDPR) may need to be updated to address the challenges posed by quantum computing.
Ethical and Regulatory Questions
Integration of quantum computing into cybersecurity raises significant ethical and regulatory issues. Key concerns include data privacy, surveillance, and potential misuse of quantum technologies.
Data Privacy and Surveillance
Quantum computing could impact data privacy and increase surveillance capabilities. Robust data protection measures are essential to mitigate these risks.
Regulatory Frameworks
Existing regulations, such as the EU’s General Data Protection Regulation (GDPR), may need updates to address quantum computing challenges. Development of new regulatory frameworks and policies is crucial to manage these concerns.
Ethical Implications
Ethical considerations are vital in the development and deployment of quantum technologies. Focus on preventing misuse and ensuring responsible use of quantum computing in cybersecurity.
Conclusion
In conclusion, quantum computing represents both a significant threat and a powerful tool for cybersecurity. While it has the potential to break traditional cryptographic systems, it also offers new methods for secure communication and data protection. This section will summarize the key points discussed in the article, highlighting the importance of proactive measures in preparing for the quantum era. It will also consider the future of quantum computing in cybersecurity, emphasizing the need for continued research and collaboration to address the challenges and opportunities presented by this transformative technology.